|
| | | | | |
|
|
|
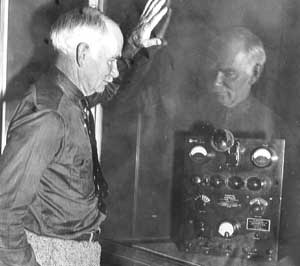 |
Forest
|
|
DOCUMENTS |
|
|
| | | | | | | | | | | | | | | |
|
| |
|
|
| Scientific American Supplement No.
1665, November
30, 1907, pages 348-350: |
|
|
|
T H
E
A U D I O
N.--I.*
A N E W R E C E
I V E R
F O R W I R E L E S S T E L E G R A P H Y.
B
Y L E E D
E F O
R E S T.
|
|
|
|
THE story of
the development
of a device of a distinctively new order, from its first inception to
its
practical reality, adds a human interest to its description which is
perhaps
too often lacking among scientific records. In 1900 when I was
beginning
experiments on the electrolytic responder, it was my good fortune to
have
to work upon it at night in my own room, at a table beneath a solitary
gas burner with Welsbach mantle. My source of Hertzian waves was the
discharge
of a small induction coil placed in an opposite corner and set into
operation
by a key closed by pulling a string.
One night I noticed to my surprise a decided
diminution
in the light from the incandescent mantle whenever the coil was
sparking.
The constant recurrence of this effect induced me to investigate. By
proper
adjustment of the inflow of gas and air to the burner, an almost
complete
extinction of its light was obtained during the sparking of the coil.
Another
adjustment even allowed an increase of the light above normal. For
several
days I was elated over the tremendously sensitive and altogether novel
type of Hertzian-wave responder thus accidentally discovered. But alas
for the over-sanguine spirits of the young investigator! When I thrust
my induction coil into a closet and closed the wooden door, thus
shutting
off the sound of its vibrator and spark, my gas light ceased to
fluctuate.
I found I had merely discovered an extremely
responsive
form of the sensitive gas flame, and that a bunch of jingling keys or a
smart clapping of the hands were almost as efficient generators of
these
Hertzian waves as was my induction coil. To hopes unrealized this was
indeed
the "light that failed."
But the few days of illusion had set me thinking.
Here in the flame around this incandescent mantle was matter in a most
mobile, tenuous state, extremely sensitive to sound and heat
vibrations,
infinitely more delicate than any arrangement of solid or liquid
particles.
Why should it not then in some phase or fashion respond to the Hertzian
vibrations also?
Unable to dislodge this conviction from my mind,
I began later to search for the genuine response to electric vibrations
in the gas flame. I found the conductivity of the incandescent mantle
surprisingly
small, however, for any voltages which would be practical in a wireless
receiver.
By soaking the mantle in a potassium or sodium
solution
and drying, I was finally able to pass a small current from a dozen dry
cells through the flame surrounding it, using two platinum electrodes
with
a telephone receiver in circuit, and get a faint response to the
genuine
Hertzian wave. The discovery that the effect predicted was actually
present
was intensely gratifying.
Experiments followed with the Bunsen burner and
other forms of flame. In the coal-gas flame the exterior luminous
portion
is positively electrified, the interior negatively. To render these
flames
sufficiently conducting, salts of the alkali metals were introduced. Of
these the caesium, potassium, and sodium salts are the most conducting,
and in the order named. These salts were either injected into the
flames,
as solution, or preferably put in a little platinum cup held in the
luminous
part of the flame and made the cathode of the telephone circuit.
A platinum wire or disk held about 2 millimeters
above this cup acted as anode. The antenna and earth connection, or the
two terminals of the oscillating receiving circuit, were connected to
these
platinum electrodes. An electromotive force of 6 to 18 volts, supplied
by a battery of dry cells, was sufficient to give a current of several
milliamperes through the colored flame.
This early form of audion, the flame receiver, was
remarkably sensitive to weak high-frequency oscillations. The sound
heard
in the telephone was an exact reproduction of that of the transmitter
spark,
in pitch, variation of intensity, etc.
It was observed that the increase of current with
electromotive force did not follow Ohm's law; a saturation value of the
current was observed. Wilson has found that the maximum current which a
salt vapor in a flame can carry is equal to the current which if passed
through an aqueous solution of that salt would electrolyze the same
quantity
of the salt as was imparted during the same unit of time to the heated
gas.
Beyond this saturation value the current will not
rise until the electromotive force is great enough to enable the field
itself to ionize the gas: that is, until the velocity imparted to the
negative
ions by the field is sufficient to enable them to separate the gas
molecules
with which they collide into positive and negative ions.
The conduction through flames under the conditions
I am describing is due chiefly to the negative ions generated, and
these
are chiefly in the vicinity of the metallic cathode. It is necessary
that
the alkali vapor comes in contact with the glowing metal. The increase
of conductivity of a flame by the addition of a salt may amount to
several
hundred per cent, and is due not to the presence of the metallic atoms
in the flame itself, but to the increase in ionization produced by the
salt at the electrodes, notably the cathode.
The velocity of the negative ions in flame at
atmospheric
pressure increases rapidly with the temperature. Thus at 2,000 deg. C.
their velocity is approximately forty times that at 1,000 deg. C. At
1,000
deg. C. the ratio of velocity of negative ions to positive ions is
calculated
as 26 / 7. At 2,000 deg. C. this negative ion velocity is about 1000
cm./sec.
for a potential gradient of one volt per centimeter.
Now suppose the average velocity of a negative
corpuscle
to be proportional to the electric force; this velocity, for a
potential
drop of 10 volts between the electrodes as I use them, is of the order
required to traverse the distance between the incandescent body and the
platinum anode during the time of one-half the wave period of the
electrical
oscillations ordinarily used in wireless telegraphy.
I shall return later to the bearing which this fact
has upon a suggested explanation of the effect of the Hertzian
oscillations
upon the gas receiver.
On account of the ionization of the gas near the
incandescent metal, and the greater velocity of the negative over the
positive
ion, it is to be expected that even if no external electromotive force
be applied to the electrodes, and one of these be relatively cold, a
current
will pass along a wire connecting the two electrodes, whose direction
is
negatively from the hotter to the cooler body in the flame. In other
words,
the colder body will be the anode, positively charged.
Now, if the Hertzian oscillations traverse the hot
gas, the momentary potentials thereby impressed upon the moving ions
will
conceivably interfere with their motions, or with the rates of
recombination
between the positive and negative ions, and thus effect the current
flowing
through the wire. A telephone connected between the electrodes
indicates
that changes of a surprising amount in the momentary potential
difference,
or flux, across the electrodes are effected by the high frequency
oscillations,
even when no external battery is applied.
When a battery of from six to twenty dry cells is
connected across the two electrodes, the positive terminal to the
cooler
electrode, the potential current curve for the conductivity of the gas
it at first approximately a straight oblique line, the current through
the flame increasing with the electromotive force.
Soon, however, this proportionality of current and
voltage ceases, and a stage of saturation is reached where there is no
appreciable increase of current with increase of voltage. But when the
potential difference is raised sufficiently to ionize the gas, a stage
is reached where the current increase is far more rapid than that of
the
potential difference. This last potential gradient depends upon the
pressure
of the gas; it is directly proportional to the pressure. This is given
by Thomson as about 30,000 volts per centimeter for atmospheric
pressure;
but with incandescent gases in an inclosed vessel at one millimeter
pressure
a gradient of 40 volts per centimeter is sometimes sufficient to
produce
this critical stage.
In the case of the flame, the distances between
the electrodes figure very little in the amount of flowing, the
potential
drop, or the sensitiveness to Hertzian oscillations, because most of
the
ionization at low voltages takes place at the electrodes.
The size and shape of the electrodes are of small
moment. I prefer a trough anode 1 centimeter long by 2 millimeters
wide,
holding the potassium salt, as cathode, and a small platinum wire
parallel
thereto and held 2 to 10 millimeters above it as anode.
The trough electrode should preferably be at the
upper tip of the oxidizing flame at its junction with the reducing
flame.
When this is made negative the current is saturated with a
comparatively
small potential difference. The gas burner itself may be used as one
electrode.
The flame must be steady and kept rich in salt. The current of
up-rushing
flame makes a rumbling noise in the telephone, which may interfere with
the detection of faint signals. This rumbling sound increases with too
great applied potentials.
The temperature, especially of the electrodes, is
an important factor. At red heat these give off positive corpuscles; at
white heat both positive and negative appear, the latter predominating.
The electrode containing the salt should always be incandescent, so
that
the excess of negative ions given off and streaming toward the other
electrode
will increase, rather than diminish the current due to the flame
itself.
The extreme sensitiveness of the flame when ionized to thermal
variations
is illuminated by the fact that a distinct response is heard in the
telephone
receiver when the mere tip of a cold pin is suddenly introduced into
the
flame. The sudden introduction of a cold body into the active part of
the
flame always reduces the response. The salt is best placed in, or on,
one
of the electrodes rather than held in the flame in an independent
receptacle,
or injected into the gas.
The applied electromotive force is a determining
factor in the sensitiveness of this receiver. The response seems
greatest
where the potential current curve is passing from the oblique to the
horizontal
portion, where the saturation value is about to be reached.
Under these conditions the sensitiveness of the flame audion is of the
same order as that of the electrolytic receiver using a glass-jacketed
electrode. The flame is not most sensitive when the flux is greatest.
There
is a close relation between the degree of heat and the critical
impressed
voltage.
Considerable difficulty was found in getting an
absolutely steady flame, even when protected by a chimney as the
slightest
air current will deflect the sensitive portion from the electrodes,
altering
the sensitiveness of response.
I next sought the phenomenon in the hot conducting
gases of the electric arc. If a wire be connected to the positive
carbon
of the arc and led through a telephone to a third electrode, platinum
or
carbon which is inserted into the border of the arc, a considerable
current
passes through the telephone. If these two electrodes are now connected
to the terminals of the receiving oscillating circuit, the conduction
of
the leak current across the gas to the third electrode is sensibly
affected
by the arriving Hertzian oscillations, if sufficiently intense. A local
battery can also be inserted series with the telephone, but the voltage
drop across the arc is usually too great to require this.
Even when the arc is fed from a storage battery
and cored carbons used, the hissing and frying noises in the telephone
(probably due to the oxidation of the terminal by the air) are
generally
too troublesome to allow a clear reading of weak signals with this form
of audion.
The principles involved in its operation are much
the same as for the flame audion. And although the intense ionization
produced
by the heat of the arc renders it extremely sensitive to slight local
variations,
its practical requirements make it less available as a wireless
receiver.
Inasmuch as the gases ionize more readily at lower
heats and are in their most mobile, delicate, and sensitive conditions
in vacuum, it seemed to me certain, after experiments with the flame,
that
the attenuated and ionized gases around an incandescent filament would
undergo very considerable changes when subjected to Hertzian
oscillations.
Elster and Geitel, [Elster and Geitel, Wied. Ann.,
xvi, 1882] beginning in 1882 a systematic investigation of the
ionization
produced by incandescent metals, frequently employed an exhausted glass
vessel containing an insulated platinum plate, stretched close to which
passes a fine metallic filament brought to incandescence by an electric
current.
Ordinarily at atmospheric pressures and red heats
a positive charge was produced upon the plate, of the order of a few
volts.
This potential increases until the wire is at a yellow heat. As the
wire
gets hotter the potential decreases, until at a bright white heat the
potential
of the plate is very slight. Diminishing air pressure has but slight
effect
upon the plate potential until very high exhaustions are reached, when
this potential begins to diminish and may even change sign, and as the
exhaustion proceeds may reach a very large negative value. This
pressure
where the plate charge changes sign depends upon the temperature of the
filament, being higher at higher temperatures.
Long-continued heating and expulsion of gas from
the incandescent metal play a considerable part in the electrical
phenomena.
Long-continued incandescence favors the negative electrification of the
plate. The presence of oxygen aids in the carrying off of a negative
charge,
thus producing negative electrification around the wire; hence the
action
of oxide of metal on filaments tends to increase the discharge of
negative
electricity. But oxygen also hastens the disintegration of the
filament.
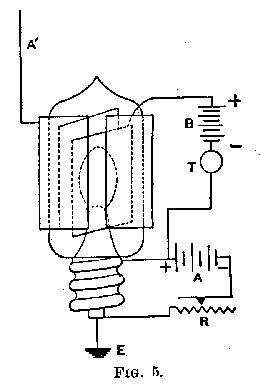 |
|
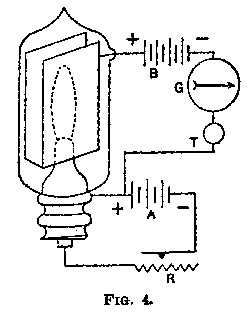 |
Gases which are
dissociated by heat
conduct on quite
a different scale from those like air, hydrogen, or nitrogen. Examples
of such are the vapors of iodine, bromine, chlorine, potassium, etc.
These
furnish a much larger supply of ions than the others. This dissociation
occurs chiefly where the gas is in contact with the glowing electrodes.
Of the metals, sodium and potassium have the highest conductivity under
the above conditions, for the emission of negatively electrified
corpuscles
from sodium atoms occurs even at low temperatures; and I have used
carbon
filaments coated with a potassium compound. The conductivity of cold
mercury
vapor does not seem greater than that of air.
With hydrogen, the plate becomes negatively
electrified
even at atmospheric pressure; and when the filament is carbon instead
of
platinum the electrification on the plate is always negative. This
means
that the gas will discharge the plate it positively electrified; that
is,
a positive current will pass from the plate to the filament in the gas.
The electrification produced in the neighborhood
of an incandescent wire is a complicated effect; it depends on the
temperature
and nature of the filament, and on the nature and pressure of the gas.
It furthermore depends upon the electric and magnetic forces to which
the
vessel is subjected; and I have found that the shape and area of the
plate
or plates, the condition of its surface and edges, as well as its
distance
from the filament, are very important factors.
If the metal plate be connected by an outside wire
to the positive terminal of the hot filament, a leak-current from the
plate
to the filament through the gas will be set up, as Elster and Geitel
first
found, passing mainly to that portion of the filament near its negative
terminal. If the resistance of the lamp filament and the lamp's voltage
be high, a very considerable leak current may thus be set up.
A battery of from three to eighteen dry cells is
connected between the positive end of the filament and the platinum
plate W,
the latter being connected to the positive pole. The saturation current
increases rapidly with the heating current through the filament, which
also increases the velocity of the negative ions, as does also an
increase
in the applied electromotive force between plate and filament.
The rate of discharge of negative electricity from
glowing carbon greatly exceeds that from platinum, while that from
tantalum
and other of the newer filaments, given the same heating current,
surpasses
the rate of discharge from carbon.
Thomson states the equation connecting the
saturation
current with the temperature as ( I = Aؽe-b
/ Ø) where Ø is the absolute
temperature, A
and b are constants.
At 2,000 deg. C. this rate of emission from a
platinum
wire in high vacuo amounts to 0.1 ampere per square centimeter of hot
surface.
For carbon this current can equal several amperes per square centimeter
surface. In the audion the flux current ordinarily ranges from 1 to 5
milliamperes.
The metal dust, or even vapor, from the incandescent
filament may play a part in the phenomena, but not a controlling one.
Thomson
has shown that the value of e / m for the
carriers
of electricity in the type of exhausted vessel described is the same as
its value for the carriers of the negative electricity in the cathode
rays,
or in the discharge of negative electricity from metals illuminated by
ultra-violet light. In fact, in many ways the behavior of the audion,
notwithstanding
the extremely low potentials used, is very similar to that of a
cathode-ray
tube; and in one or two small pea lamps where the anode disk was close
to the bend of the filament I have actually obtained, at only 22 volts,
a blue-white beam of light playing between the filament cathode and the
anode. Upon the approach of a powerful magnet this beam could be
concentrated
and deflected. A great increase in the current through the telephone
marked
the formation of this beam, and a violent hissing or squealing sound
began
when the magnet was approached.
The corpuscles at the filament are attracted by
the metal of the filament, and to escape into the surrounding space
they
must be given sufficient kinetic energy to carry them through the
surface
layer where this attraction for the carriers is appreciable. Thus, as
the
temperature of the filament increases, a larger number of the carriers
can escape from the wire. But the saturation values of the flux current
do not depend upon the velocity of the ions, but only upon the number
of
ions produced in unit time at the surface of the hot metal.
The source of ionization is confined to the gas
immediately surrounding the filament. The velocity of the ion at any
instant
is dependent on its distance from the filament, because the temperature
is not uniform between filament and plate. The ratio of the velocities
of negative to positive ions varies greatly with the temperature: thus
they are given as 1,000 to 62 at 2,000 deg.
This fact explains why the positive conductivity
of the gas in the vessel is almost entirely from the cold to the hot
electrode
in the gas, and not in the reverse direction; and why this
unidirectional
quality is more marked for higher temperatures of the cathode, the
anode
being kept cold.
In the form of audion illustrated in Fig. 4, I
employ
two platinum wings parallel to the plane of the bowed filament and
about
2 millimeters on either side of it. These wings are soon coated with an
iridescent deposit from the metal filament, especially at the portions
opposite to the negative half of the filament. They become quite hot at
this short distance, but not sufficiently hot to take part in the
ionization
of the gas.
When connected in the oscillation circuit as shown,
properly attuned to the receiving electromagnetic impulse from the
antenna,
the audion under proper adjustment of heating current and battery B
I find the device extremely closely tuned with the
syntonizer, for its operation seems to be dependent upon the sum total
of the energy received from the complete wave train rather than upon
the
maximum first impulse of the train. In other words, while instantaneous
as far as our senses or instruments can perceive, its action is
sufficiently
sluggish to be determined by the additive effect of the entire received
electro-radiant energy through a short time interval.
When the filament is first lighted, an appreciable
interval, about one-quarter second, elapses before the full
sensitiveness
is established. Before the flux reaches a steady state there is a
period
during which the number of ions is steadily increasing. As a result of
the colliding of the initial ions with the gas molecules the number of
ions and the current rapidly increase, until an equilibrium is finally
attained.
The audion, to a greater extent than any other
responder,
is self-tuned. I mean that by regulating the heating current, the
potential
between wing and filament, or the distance between these, the audion
can
be made to a great extent selective per se to certain received
impulses.
And the determining factor here is not merely the frequency of the
electrical
oscillation; the spark frequency, or factors determining the total
amount
of energy received during a very brief unit of time, determine to an
extent
the amount of its response. Thus with 12 volts across it, it may give a
loud response to a transmitter A; and with 10 volts "bring in"
another
transmitter B to the almost complete exclusion of A,
although A
and B are of equal power and of approximately the same
wave-length,
but differing considerably in spark frequency. Similar discrimination
can
be produced by adjustments of the heat of the filament, which also
governs
the amount of flux through the gas. This flux is generally reduced when
the audion is placed in a strong magnetic field, especially when the
lines
of force pass through the gas parallel to the plane of the wings, at
right
angles to the electric field. By this means also a tuning can be
effected.
Again, it is not necessary to connect the anode
to a terminal of the oscillating circuit. One terminal may be attached
to a metal sheath or ring surrounding the glass vessel, thus forming a
condenser with the filament or the conducting gas within the tube. In
this
case the adjustment of the syntonizer is generally different from that
required for the same oscillation frequency, when the interior wing is
directly connected in the oscillation circuit. In this condenser
arrangement
also the sound heard in the telephone changes its quality to an
extraordinary
degree, being of a dull muffled nature rather than sharp and staccato.
Signals of this quality are sometimes much more readily distinguished
from
the "static" disturbances which so frequently render wireless signals
difficult
to read. The operator has thus a ready means of changing the quality of
the received signals to suit the conditions. This latter type or audion
is the one I have found most serviceable in practice.
The audion may even be placed in the space between
two plates of an air condenser in the oscillating circuit. A
flat-walled
type of tube is preferred for this arrangement. Again, the electrical
oscillation
may be led through a coil of wire wound around the outside of the tube
(Fig. 6), and not through the audion at all, or through a flat coil
brought
up close to the tube, with its axis perpendicular to the filament. In
this
arrangement it is chiefly the electromagnetic component of the passing
oscillation which affects the motion of the ions within the vessel. The
ions are readily influenced by a magnetic field.
By shifting the syntonizer connections from the
wings to this helix, I have been able to cut out completely signals
from
a transmitting station so near as to baffle all attempts with the
ordinary
tuner methods, and to bring in other relatively faint signals.
The audion has the further advantage of entire
absence
of adjustment in the receiver itself. It gives no evidence of fatigue
under
any conditions of use. Furthermore, it requires no protection from the
violent impulses of the transmitter at its own station, whereas the
sensitiveness
of the electrolytic receiver is completely destroyed by one such
violent
impulse, unless its small electrode is protected by a shunting switch.
I have arrived as
yet at no
completely satisfactory theory as to the exact means by which the
high-frequency
oscillations affect so markedly the behavior of an ionized gas. Fleming
points out that when the cold plate of the Elster-Geitel tube is
connected
to the positive end of the filament, and the two put in a high
frequency
oscillation circuit, only the positive half of the oscillation can pass
from the plate to the filament across the gas. He uses this principle
to
rectify the Hertzian oscillations, and applies the unidirectional
currents
of the oscillations themselves to operate a sensitive galvanometer or
direct-current
instrument, for quantitative measurements over short distances.
When an independent external source of electromotive
force is applied, in the manner I have described, the action becomes
quite
different. It then operates as relay to the Hertzian energy
instead
of merely rectifying this energy so that it can be used directly to
give
the sense signal.
The audion, therefore, is tremendously more
sensitive
and available in practical wireless. A sensitive direct-current
instrument
in the B circuit shows steady deflection varying not a whit, by
increase or decrease, during the reception of strong "wireless"
signals.
An electrolytic receiver or "polariphone" under similar conditions
would
cause a great deviation in the deflection of a milliammeter, although
the
signals in the telephone with the electrolytic are not so loud as with
the audion.
I have connected two audions in series in opposition
in the oscillating circuit each with its separate beating circuit, and
still heard the signals in the telephone connected to the second audion
equally well, whether the wing in the first be connected to the wing or
to the filament of the second.
When one of the tubes is unlighted or if lighted
its B circuit is open, no high-frequency impulses pass through
it
unless the wing and filament are very close together. When cold it acts
merely as a condenser whose armatures are the wings and filament and
whose
capacity is extremely small.
(To be continued.) potential is extremely
sensitive, giving response In the receiving
telephone
several times as loud as any other form of wireless receiver when
subjected
to the same impulses. It is, however, less sensitive to atmospheric or
static disturbances, which are strongly damped or aperiodic.
|
|
|
|
|
|
|
|
|
|
|
| Scientific
American Supplement No. 1666,
December 7, 1907, page
354-356:
T H
E
A U D I O
N.--I
I.*
A N E W R E C E
I V E R
F O R W I R E L E S S T E L E G R A P H Y.
B Y L E E D
E
F O R E S T.
Concluded from Supplement No. 1665,
page 350.
I HAVE laid
considerable stress
upon the potential gradient or "variation" layers which exist near the
surface of the electrodes when the external applied electromotive force
is considerable, for the reason that their existence serves to play a
very
important rôle in the response of the audion to minute high
frequency
oscillations.
If the velocity of negative ions is very large
compared
to that of the positive ions, the curve representing the distribution
of
electrical intensity between the two electrodes is represented by the
following,
which is typical.
When ions of both signs are present in the gas and
when the electric field is so strong that most of the positive ions are
driven from the anode and the negative ions from the cathode (the
filament),
we will have an excess of cations in front of the anode and of anions
surrounding
the cathode.
It is seen that the variation in potential lies
chiefly in the thin layers of gas in front of the two electrodes. It is
convenient to speak of these regions as the "variation" layers. As
Thomson
points out, in passing from the inside to the outside of the layer of
ionized
gas we have to pass across a layer of electricity. This will produce a
discontinuity in the electrical intensity equal to 4 pi times
the
surface density of the electrification.
There may thus be a great difference between the
electric intensity inside the layer and that just outside. The
potential
drop across the layer is proportional to the square of the current; the
falls of potential at the positive and negative electrodes are
proportional
to the squares of velocities of the positive and negative ions; and the
velocity of the ions is proportional to the electric force acting upon
them.
These variation layers at the electrodes of the
audion make still more striking its similarity with the cathode-ray
tube.
In the cathode tube a sudden drop in potential called the "anode fall
of
potential" occurs quite close to the anode; and in the layer called the
Crookes dark space, or cathode dark space, there is a still greater
fall
in negative potential. But the voltages here are enormously higher than
those in the audion. As the gas pressure in the cathode tube
diminishes,
the dark layer, or the cathode drop layer, becomes broader. D =
a
+ ß y; that is, the width of the dark space is
proportional
to the mean free path of the molecules, beyond a certain distance a
in front of the cathode. Schuster found that the thickness of the
cathode
drop layer increased slightly with the current passing through the gas;
but Wehnelt found just the reverse. Both may be correct on different
sides
of some particular value of the current for which the width of this
space
is a minimum. This is interesting in view of the fact that there is a
certain
current flux across the gas of the audion for which the response of the
Hertzian oscillations is maximum; supposing this response is maximum
when
the width of the variation layer around the filament is minimum.
Within the cathode layer there exist only negative
ions, these being shot off from the cathode. Right outside of this, in
the region called the "cathode glow," ionization of the gas from
collisions
with these negative ions begins, and the width of the cathode dark
space
is about the range of the "mean free path" of the ions.
If a similar state of affairs exists around the
filament of the audion, and if this mean free path of the cations
coincides
with the excursion of the corpuscles during one half the oscillation
period
of the impressed Hertzian vibration, we might expect under these
conditions
a maximum effect of response to oscillation of the particular
wave-frequency.
Or a similar effect might be expected if the excursion in question is
that
of an ion from the cathode across the gas up to the layer surrounding
the
anode.
The extent to which the sensitiveness of the audion
is governed by a very slight change in the heating current, or in the
potential
drop across it, seems to lend plausibility to such an explanation. And
it has been shown that in conducting flames at atmospheric pressure, a
negative ion acting under a potential gradient of 10 volts per
millimeter
would travel approximately 1 millimeter, or a commonly found distance
between
the electrodes in the audion, in 1 / 1,030,000 part of a second, which
time-interval is of the order of one-half of the wave period of some of
the longer oscillations used in wireless telegraphy. For reduced gas
pressures
the natural excursion of the ion would be more rapidly accomplished,
but
its velocity can be governed within wide limits by regulating the
applied
electromotive force. When we send more current through the filament we
increase the potential difference between filament and anode as well as
increase the heat. Both changes act to increase the ionic velocity.
In Humstedt's experiments where a cathode-ray tube
was exposed to high-frequency oscillations, the width of the cathode
drop
layer, or dark space, diminished as the frequency of the oscillations
increased;
as if there might be some connection between the period and the time
involved
in the immigration across. And many facts observed in connection with
the
audion otherwise difficult to explain tempt one to suppose that here
the
degree of response is connected with the relation between the product
of
velocity of the ions by the distance between the electrodes and the
period
or half-period of the electrical oscillations received.
When the anode consists of two parallel plates
instead
of a cylinder there will be a maximum of positive electric density
along
their vertical edges. The more intense parts of the electric field will
involve the larger number of ions, and on the anode these will
generally
be located at the vertical edges of the parallel plates, provided these
are not too far from the filament.
With this type of anode a peculiar and sudden
inflection
point in the current-flux diagram, as the heating current is gradually
increased or decreased, is noticed. The flux goes on increasing, then
suddenly
drops back to a lesser value; at the same time a click is heard in the
telephone in the B circuit. Then as the heating current is
still
further increased the B flux is again increased. These same
cusp
points in the curve are obtained if the A circuit be kept
constant
and the B voltage is increased instead. Similarly, a click is
heard
when the flux current is being reduced from a higher value, only the
location
of the cusp on the curve of decreasing current is not coincident with
but
lags behind that observed when B is being increased. This
second
cusp point shows a sudden increase in the flux current, when
the
critical point is reached, to a value previously passed through.
Naturally
the sharpness of these cusp points can be smoothed out or quite
obliterated
by putting impedance in the B circuit in series with the
telephone.
The diagram (Fig. 9) shows the relative magnitude
of these sudden alterations in the flux current obtained with a certain
sample audion, and also the decided hysteresis effect, showing how the
actual B
These hysteresis curves are always obtained even
though the anode is in the form of a cylinder or flattened cylinder
without
the vertical edges; but the reactive cusp points in the curves
are
never obtained save with two plane anodes connected together.
Zeleny [Zeleny, Physical Review, vol. xii, 1901]
has found a similar very curious hysteresis effect in the currents
obtained
from the ions from a platinum wire when heated and exposed to
ultra-violet
light. When the metal was cooling these currents were greater than
those
for the same temperature when the metal was being heated. In this case
heating the wire produces some change in its surface, possibly in the
amount
of gas condensed thereon or absorbed by it, from which it recovers very
slowly.
As B voltage is increasing and A
current
is increased and decreased, I find that the points at which the cusps
occur
on the increase and decrease A-B curves coincide more
and
more nearly, and at the same time these cusps become less and less
violent.
The hysteresis effect is less pronounced as the B voltage is
increased.
As shown in the curves for a large B flux the two A-B
curves for increasing and decreasing A current coincide almost
exactly
until BB flux, again diverge, and then
coincide
once more near their origin. These curves were all taken with audions
of
the double-wing type, which feature may account for some of the very
peculiar
characteristics observed.
The filament is always at some part nearer to one
wing than the other: Hence the B flux is chiefly concentrated
on
this wing or portion of wing, beam of cathode rays. We may suppose that
as the B voltage is increased, as when more heating current is
passed
through the filament, the flux is increased and spreads out over this
wing
until a new sheaf or "ray" of ions, starting off from the filament from
another part or in a new direction, suddenly leaves that and takes by
preference
a shorter path to the opposite wing. We would suppose that a new path
thus
taken would first be located on one of the vertical edges of the wings
parallel to the filament.
This sudden diminishing of the intensity or density
of the original beam of ions may be accompanied by a decrease in the
velocity
of propagation of the ions, and thus the resultant flux be actually
less
than before. The reverse operation will occur when the B flux
is
being decreased from a high value.
When the anode consists of one wing only, no such
reverse cusp-points, or reversals of the flux increment, have been
obtained.
With a single-plane anode, however, there is found a point at which the
flux increasing assumes a sudden increase in magnitude, presenting an
abrupt
rise in the otherwise smooth flux voltage curve; and the reverse when
the
current flux is being decreased.
These effects seem to relate to the increased values
of the positive variation layers along the vertical edges of the anode
which parallel the filament. The distribution of the charge upon the
surface
of plate may be described as analogous to that of a film of liquid
which
coalesces and is heaped up along the edges, and from which, when the
liquid
is by any means drawn away, there is a sudden recession: the liquid, on
account of the surface tension, letting go or taking hold of the edge
all
at once.
It is significant that just at a cusp point the
sensitiveness of the audion to the hertzian oscillations attains a
marked
maximum. Under the critical conditions then, obtaining the slightest
change
in the applied electromotive force is accompanied by relatively great
changes
in the B flux.
In framing any theory of the action of electric
oscillations in the audion a variety of complex contradictory phenomena
are met with, exceedingly puzzling to explain. An example is the fact
that
a continuous current instrument either in the A or the B
circuit shows absolutely no change of deflection either of increase or
decrease, when B is large and the audion in its most sensitive
condition.
If only the positive halves of the oscillations pass from anode to
filament
these should increase the reading of a milliammeter in the B
circuit
during the passage of a long series of wave-trains of sufficient
intensity.
Or else the negative halves of these oscillations might be expected to
diminish to a greater degree the positive charge on the anode, and
result
in a diminution of the B circuit. Or if both of these acted
equally
and oppositely no signal would be obtained at all, for the telephone
diaphragm
is utterly incapable of following such rapid increase and decrease in
the B
current, even if its impedance would allow these pulsations to pass
through
the circuit. Neither would the ear detect such vibrations.
If on the other hand the integrated effect of a
complete hertzian wave-train were either to increase or decrease the B
flux, a long succession of such effects, all of which must be of the
same
sign, ought to cause a change in the needle's deflection, as when a
long
Morse dash is sent out from the transmitting station. We have no reason
to suppose that one wave-train, the result of one spark, would produce
a momentary increase in the B flux, indicated by a click in the
telephone, and that the next succeeding wave-train from the next spark
would cause an opposite increase in B flux, and another
similar
click in the telephone. Such action would of course explain why a loud
sound in the telephone might not be accompanied by any change in the
sluggish
ammeter reading, similar to the case with the magnetic detector.
The following explanation of the phenomena which
seems to account for many of the peculiarities of this paradox has been
suggested. It should be remembered that if the negative half of the
electric
oscillation can not pass through the gas from cold anode to the
filament
the audion electrodes during that period will act merely as the two
armatures
of a condenser. Even when close together, their mutual capacity, when
the
gas is cold, is exceedingly small, and only a very small positive
charge
can be held bound on the filament; or if there are sufficient free
positive
ions in the hot gap the complementary positive charge will be held just
on the outside of the "variation layer" at the anode.
The fall of potential across the variation layers
at anode and cathode are proportional to the squares of the velocities
of the positive and negative ions; and the ionic velocities are
proportional
to the electric forces acting upon them. Supposing then that during the
positive half of the electric oscillations the velocity of the positive
ions is increased at the anode layer, during the other half period the
velocity of the negative ions is increased, due to the changes in the
electric
force acting upon them. Then regardless of the sign of the change of
the
velocities the potential drop across the variation layers (which varies
with the square of these changes) will be increased during the entire
passage
of the oscillation train.
The layer will act during this interval like a
condenser,
the potential drop across which is momentarily increased, which
momentary
increase will disappear with the passage of the wave-train. It will be
as though the plates of a charged air condenser were suddenly further
separated
and then brought suddenly back to their normal positions; or as though
the specific inductive capacity of the dielectric were decreased and
then
increased. This operation being repeated for every spark at the
transmitter
a listener in the telephone in the B circuit will hear a sound
whose
pitch is exactly that of the spark, while an ammeter in that circuit
will
show no variation in its deflection.
As the fall of potential across the variation layers
is proportional to the square of the current passing to that of the
impelling
electric force, it is readily understood how, by regulating the heating
current and the B voltage, an optimum value of the electrode
drop
may be obtained for which the effect from any given received impulses
will
be a maximum. Also how by varying the distance between the electrodes
the
sensitiveness of response may be regulated.
Thompson states that the current between two plates
for a given difference of potential varies inversely with the cube
difference
of the distance between the plates, up to the saturation-current stage.
But in the case of the audion where the cathode is an incandescent
filament,
the law seems to be quite different. Thus for two anodes of equal area,
one approximately four times as far from the filament as the other, the
two currents were as 21 to 8. The flux here varies more nearly with the
inverse distance.
The potential difference required to produce
saturation
is proportional to the square of the distance between plates and to the
square root of the intensity of ionization. This latter depends on the
temperature of the filament.
In the case of the parallel plates, only one of
which is incandescent, or if both are heated but below yellow heat so
that
only ions of one sign (positive) are present and carrying the current,
then this current as Thomson shows is:
i = 9 R V ² / 32 pi d
³
where R is the velocity of the ion under unit electric force, V
the potential difference, D the distance between the two
plates.
According to this formula the current varies inversely as the cube of
this
distance. But this formula will hold only when R is independent
of X, which it will not be when the temperature through the
space
is not uniform. It holds also only for currents that are small compared
with their saturation values, for the saturation currents depend not
upon
the velocity of the ions but upon the number of ions produced in unit
time
at the surface of the hot electrode. But in the case of the audion with
small potentials, the closer the electrodes are together the more
rapidly
will the B current increase as the potential drop is increased.
The trajectories of the ion are shorter and they therefore undergo
fewer
collisions, reunions, and retardations when the electrodes are close
together.
In an audion where the anode is far from the filament the saturation
current
is not attained with the B
In some cases a remarkable lag or "creeping effect"
is observed at this saturation stage. As shown in the curve, the
milliammeter
needle crept slowly up after B was raised to 14 cells, from 18
to
26 divisions. The current flux required something like 15 seconds in
this
instance to attain its full value. The filament in this case may have
been
undergoing some change which caused it slowly to discharge more and
more
corpuscles until that stage was reached where the recombination of
oppositely
charged ions in the gas exactly equaled the output of negatively
charged
ones from the incandescent surface. Sometimes this creeping is
accompanied
by a loud frying sound in the telephone.
current lags behind the increasing or decreasing
electric
field which produces it. This hysteresis effect is very like that
obtained
when the molecular structure of iron is altered under a changing
magnetic
field. Doubtless it is here due to a reluctance of the ions to
accommodate
their paths and velocities to the impelling electric forces; and the
area
included between the two curves represents the work lost in
accomplishing
this conformation. flux is reduced to a certain amount. They may again
cross
each other at a lower point of voltages used with the audion in
wireless
telegraphy. We sometimes have instead its inverse counterpart, a
saturation
voltage, so to speak. As shown in the curve, at potentials from 10 to
18
volts a slight potential increment is accompanied by a very large
increase
in flux. And within these limits the sensitiveness of electric
oscillations
may be a maximum. The cusp points when present are generally found near
these points of inflection in the flux-voltage curves.
MAGNETIC EFFECTS.
Thomson shows that at
low gas pressures and high
ionic
velocities the ions, when placed in a strong magnetic field, will
travel
along the lines of strong magnetic force; but when the product of
velocity
and field is small the ion moves parallel to the electric force. If
both
magnetic and electric forces are uniform, the ions both positive and
negative
will move in the same direction and perpendicular to both E and
H.
When the electric field is not uniform but radiates from a point, and
the
magnetic field is uniform, the ion will describe a spiral traced on a
cone
of revolution whose axis is parallel to the magnetic field.
If the directions of EH
If the electric field is not uniform (and it is
not in the audion where the negative charge is located on the small
cylinder
of the filament instead of on a plane surface) the paths of the ions
will
not be cycloids, but in any case the ions will be turned back by the
magnetic
field after traveling a certain distance d, from their source.
Thus
they will never get farther than d from their source.
When the lines of magnetic force are perpendicular
to the discharge in the cathode-ray tube, the magnetic field at all
pressures
retards the discharge and diminishes to a considerable degree the great
drop in the electric force which occurs in the negative glow.
In general it can be assumed that in a strong
magnetic
field the ions tend to follow the lines of magnetic force. The smaller
the velocity of projection the more nearly does the path of the ion
coincide
with a line of magnetic force. In cathode-ray tubes the boundary of the
negative flow may coincide with the lines of magnetic force.
In the case of the audion if the lines of a strong
magnetic field pass through the gas parallel to the plane of the
anodes,
a marked reduction in the flux is obtained, sometimes amounting to 20
per
cent. This effect is greater when the south pole of the magnet is
nearest
that leg of the filament which is attached to the negative terminal of
battery A. The negative charge on this leg is of course greater
than on the other, for the negative charge on the other is the
resultant
of the negative potential of battery B and the positive
potential
of battery A. And when the lines of magnetic force are so
directed
as to tend to sweep some of the negative ions off from the parts of the
anode nearest to the filament leg which carries the greater negative
potential,
the reduction of the flux across the gas will be the greatest possible.
Hence the magnetic polarity observed.
If the filament extend above the top of the anode,
say for 0.5 centimeter, then a magnetic field parallel to the filament
legs may tend to force certain lost ions into a downward trajectory so
that they will strike upon the anode instead of passing off above it.
In
this case only is an increase in the B flux observed as a
magnet
is brought up to the audion.
In general the flux will be diminished by the
magnetic
field. When the magnetic lines pass perpendicular to the plane of the
wings
the negative ions which are traveling in the direction of the magnetic
force, from filament to wing, will be accelerated, but those originally
traveling out from the filament in the opposite direction will be bent
around or deflected from their direct paths; so the resultant will be a
decrease of the total current flux.
When the field is intense, a marked frying or
hissing
sound in the telephone is heard, especially with the two-wing anode,
and
when the magnetic force is parallel to their plane and thus affecting
mostly
the ions which are streaming toward their vertical edges. In the
hissing
are parts of the arc are in rapid motion in the unstable portion around
the edges of the positive terminal. Possibly also the presence of
oxygen
the gas enters into phenomena here as it does in those of the hissing
arc.
As the magnetic field lengthens the arc so here it lengthens the paths
of the ionic discharge.
The hissing is much more violent when the surfaces
of the anode instead of being plane are punched full of little holes
whose
ragged and protruding edges offer a greatly increased opportunity for
the
ions to travel irregularly under the combined forces of the magnetism
and
of the electric charges heaped up at all such points and edges. In this
particular audion, I could get a great range of singing or squeaking
sounds
as the heating current was varied. Where the velocity of the ions is a
maximum their deflection by the magnetic field will be lessened.
If the B flux is too great to give maximum
sensitiveness of response, bringing up a magnet to the audion will
increase
the strength of the wireless signals, because of the reduction of the B
Consider the case where the electric oscillations
instead of being introduced into the audion through its interior anode
are brought up to a metal plate outside a vessel. Electric displacement
currents instead of conduction currents must then act upon the ions
within
the vessel and on the charges upon the electrodes.
Now in the case of an electromagnetic wave, where H
and E are perpendicular to each other and to the direction of
propagation,
Thomson shows that if the product of H × e is
large
(e being the electric charge on a carrier) the average velocity
of the ion parallel to the direction of E is zero, and the wave
will carry the ion along with it. When, however, H × e
is small (no external magnetic field) the effect of the Hertzian wave
will
be to superimpose on the undisturbed motion of the ion a small
vibratory
motion parallel to the electric force of the wave and thus
perpendicular
to its direction of propagation.
A very convenient form of audion for investigating
the relations which the distance, area, etc., of the electrodes bear to
its response is had by using a pool of mercury for the anode. This is
conveniently
held in one or more pockets blown in the walls of the glass vessel, and
the filament so placed as to pass closer to some than to others.
Quite frequently I obtain with this arrangement
two maxima of sensitiveness to the same transmitter, the filament
heating
current remaining unchanged; thus one maximum for B = 12 volts
and
a second for B = 18 volts. Again the sensitiveness is maximum
when
the mercury surface is as near as possible to the filament. When a
globule
has rolled out of its pocket, exposing a new surface for the anode,
sometimes
half a second elapsed before the sensitiveness is again restored. This
form of mercury tube was especially sensitive to the influence of a
magnetic
field.
The optimum or critical voltage of B becomes
less after this audion has been heated a little time, as though the
heated
mercury vapor began to act to increase the conductivity of the gas.
This
critical voltage keeps reducing as the vaporization proceeds, and with
a sudden jar on the tube I can bring this down, one cell of B
at
a time accompanied by a loud click in the telephone at each reduction.
Sometimes a similar reduction of the B flux amounting to as
much
as 25 per cent can be obtained with the double platinum wing type of
audion,
by striking it smartly; or a sudden increase in the flux may be
obtained.
The heating current when a large anode surface is
used is less than that required to produce the same degree of
sensitiveness
with a small pool of mercury as anode. In general the flux is quite
proportional
to the area of the anode, other conditions remaining unchanged. A
mercury
arc also may be substituted for the filament, but such an arrangement
is
apt to be noisy in the telephone.
When the hertzian oscillations are passed through
the filament instead of through the gas, they require to be of great
intensity
to give any response whatever. Any results from the added heating
effect
which they may contribute to the filament are quite insignificant. The
response when the audions are connected up in parallel, or series, is
always
less than for one alone.
In a tube whose two-plane anodes are fitted on
hinges
and backed with small iron disks so that their distances from the
filament
can be regulated by an external magnet, I find the response to a long
wave-length
greatest when this distance is the greatest possible; while to a
wave-length
of about one-half this, the response is decidedly better when the wings
are nearer to the filament. Of course the B flux is greater in
this
latter case, other conditions being unchanged; but the selective
quality
in this tube just described seems to be due to the regulations of the
distance
between anode and cathode rather than to other factors.
The manner in which the audion should be located
in the oscillating circuit, as well as many other considerations, show
conclusively that it is a "potential-operated" rather than a
"current-operated"
relay receiver. At the same time its advantageous sluggishness of
action,
as explained above, renders it additive in its response to the energy
of
an entire wave-train or even of a series of wave-trains. Hence its
excellent
and marked selective qualities.
A large number of experiments have been carried
out with a view to reducing the filament heat necessary to give the
inclosed
type of the audion the extreme sensitiveness which now characterizes
it.
This is now attained at normal brilliancy of the filament, or a little
below; never at excessive heats. Thus the life of an audion should be
that
of an incandescent lamp of the same class of filament and voltage.
Filaments have been coated with alkali metals or
salts, or vapors of these introduced into the tubes. Experiments along
these lines and with various dissociable gases are being pushed with
gratifying
promise of our soon being able to achieve the present marked
sensitiveness
even at red heats; or of still further multiplying the sensitiveness.
Radio-active compounds, applied for example between
juxtaposed metal disks and heated, give little encouragement. At the
low
voltages used no increase of conductivity by their means has been
observed,
although Swinton has found that a radium coated cathode in a
cathode-ray
tube has a marked action in facilitating a luminous cathodic discharge,
when the cathode is heated to redness. The mere presence of radium in
the
tube is insufficient to produce the effect.
Spontaneous ionization, that is the ionization
independent
of the electric field, as for example that produced by the X-rays, does
not increase the current flux. Only the ions produced by the electric
field
itself close to the cathode, and by the heat of the cathode, are
effective.
It is required that the audion be made with
scrupulous
care; a trace of impurity in the gas may produce surprisingly large
effects
in the potential across the variation layer. The presence of a mere
trace
of moisture may cause great difference in the behavior of a tube.
In all this work a bewildering host of new and
puzzling
phenomena is continually encountered. By its nature clean and pretty,
fascinating
in its ever new phases, gratifying in the efficiency with which it
responds
to the difficult demands of a new and intricate art, the audion
combines
infinitely delicate matter and forces, at once offering rich fields for
study to the physicist and delight to the practical man. and coincide,
the path of the ion itself is a helix of gradually increasing pitch,
with
its axis parallel to the lines of magnetic force. The radii of the
spirals
will be small compared to the length of the mean free path of the ions.
This is especially true for the negative ions, even when the motion of
the positive ions is but little affected by the magnetic field. flux.
Or if this flux be already below the optimum then the presence of
the magnet may decrease the sensitiveness. This effect may be more
pronounced
for one wave-frequency than another, in which case the audion can be
attuned
by regulating the magnetic field to which it is subjected.
* For its name,
Audion, a title as
beautiful as
it is appropriate, I am indebted to my assistant, Mr. C. D. Babcock,
who
has been of utmost service to me in the development of this device
almost
from its inception.
Copyright 1906, by the American Institute of
Electrical
Engineers, before which society this paper was read.
|
|
|